Insight
Solid State Fermentation – Part 2: Technology Design and Process Utilisation
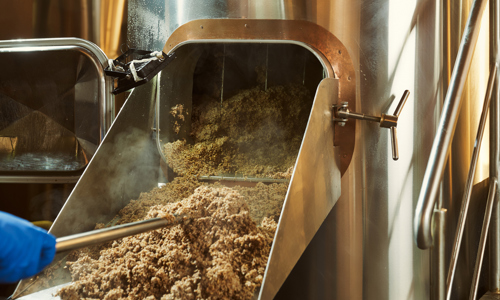
Credit: SerhiiBobyk
Insight
Credit: SerhiiBobyk
In Solid State Fermentation Part 1, we explored the foundational aspects of Solid State Fermentation (SSF), focusing on the processes involved and its growing industrial applications. With the ability to transform agro-industrial wastes into valuable products like enzymes, biofuels, and organic acids, SSF has emerged as a sustainable alternative to traditional fermentation methods.
Critical insights into microbial selection, substrate preparation, and the various stages of fermentation established the groundwork for understanding SSF's potential in biotechnology.
...
In Part 2, we move from the theory behind SSF to the technological advancements and process improvements needed for industrial scaling.
In this section, we dive into bioreactor designs, process control systems, and advanced methodologies that enable efficient heat and mass transfer, crucial for maximising yields and ensuring consistent product quality.
By addressing challenges such as aeration, temperature, and moisture management, we further explore how cutting-edge technologies are shaping the future of SSF, allowing it to meet the demands of modern industrial biotechnology.
Various bioreactor designs are used in SSF to optimise conditions for microbial growth and product formation. These include tray bioreactors, packed bed bioreactors, rotating drum bioreactors, and fluidised bed bioreactors.
Tray bioreactors:
Tray bioreactors are simple, static systems where the substrate is spread out in thin layers on trays. They are commonly used for fungal fermentations and provide good aeration and easy temperature control.
Packed bed bioreactors:
These bioreactors involve packing the substrate in a column or bed, through which air or gas is passed. They offer improved aeration and temperature control compared to traditional tray systems. However, ensuring uniform substrate distribution and preventing channelling are critical challenges.
Rotary drum bioreactors:
Rotary drums provide intermittent agitation, which enhances heat and mass transfer. They are particularly suitable for large-scale SSF but require careful design to minimise mechanical stress on microorganisms.
Fluidized bed bioreactors:
Fluidised beds use a continuous flow of air to suspend substrate particles, ensuring excellent mass and heat transfer. These bioreactors are highly efficient but require precise control of air flow to prevent particle aggregation.
Multi-Stacked Circular Tray Bioreactors:
A newly designed multi-stacked circular tray bioreactor offers improved gas exchange and moisture distribution. This design facilitates large-scale production with consistent quality and yield.
Forced aeration is a critical component of aerobic SSF processes, where the continuous supply of air ensures adequate oxygen transfer to the microbial culture. This method enhances microbial growth, metabolism, and product formation by maintaining optimal oxygen levels and removing excess heat and carbon dioxide.
Air supply systems:
Forced aeration systems use blowers or compressors to introduce air into the fermentation substrate. The air is distributed evenly throughout the substrate bed to maximise contact with the microorganisms.
Heat and mass transfer:
Aeration facilitates heat dissipation from the exothermic microbial processes, preventing overheating. It also enhances mass transfer, ensuring that oxygen reaches all parts of the substrate and that metabolic by-products like CO2 are efficiently removed.
Increased productivity:
Adequate oxygenation supports higher microbial activity and productivity, leading to increased yields of the desired products.
Temperature control:
Effective heat removal through aeration maintains optimal temperature conditions, crucial for enzyme activity and microbial growth.
Uniform fermentation:
Forced aeration ensures uniform fermentation conditions, reducing variability in product quality.
Enzyme production:
Forced aeration is used in the production of enzymes such as amylases, cellulases, and proteases, where high oxygen levels are required for optimal microbial activity.
Organic acid production:
The production of organic acids like citric acid and lactic acid benefits from forced aeration to maintain aerobic conditions and enhance yield.
Biofuel production:
In bioethanol production from lignocellulosic biomass, forced aeration helps in maintaining aerobic conditions necessary for the growth of Saccharomyces cerevisiae and other yeasts.
Temperature is a critical parameter in SSF, influencing microbial growth, enzyme activity, and product formation. Most microbial processes generate heat, which can accumulate and negatively affect fermentation if not properly managed.
To control temperature during fermentation, bioreactors can be equipped with cooling systems such as water jackets or forced air circulation to dissipate excess heat.
Continuous monitoring using sensors ensures that temperature remains within optimal ranges, typically between 25°C and 35°C, depending on the specific microorganism and product.
Ensuring uniform temperature distribution throughout the substrate can be challenging due to the solid nature of the medium, this can be mitigated by designing bioreactors with efficient heat transfer capabilities and implementing periodic mixing or agitation can help maintain consistent temperatures.
Maintaining appropriate moisture levels is essential in SSF as it affects water activity (aw), which in turn influences microbial activity and product yield.
Addition of moisture into the system can be done by periodic spraying or humidified air can be used to maintain desired moisture levels in the substrate.
Real-time monitoring of humidity levels helps in maintaining optimal conditions, typically ranging from 50% to 70%.
Achieving uniform moisture distribution within the substrate can be difficult, leading to dry spots or over-wetted areas, but it is possible create an advanced bioreactor designs and automated humidity control systems can ensure even moisture distribution, enhancing microbial performance.
Oxygen is critical for aerobic SSF processes, where sufficient oxygen levels are necessary for microbial metabolism and product formation, oxygen control can be achieved by:
Forced Aeration: Blowers or compressors are used to introduce air into the bioreactor, ensuring that oxygen reaches all parts of the substrate.
Oxygen Sensors: Continuous monitoring of oxygen levels ensures that they remain within optimal ranges, typically around 20-25% oxygen concentration.
Ensuring uniform oxygen distribution can be challenging, especially in large-scale systems, but using well-designed aeration systems and optimizing substrate packing density can improve oxygen transfer efficiency.
Carbon dioxide (CO2) is a by-product of microbial metabolism. Accumulation of CO2 can inhibit microbial activity and affect product formation.
Effective ventilation systems are used to remove excess CO2 from the bioreactor.
Adding CO2 sensors in the bioreactor design, makes it possible to have real-time monitoring of CO2 levels, which ensures that they do not exceed inhibitory concentrations, typically below 5%.
Balancing CO2 removal with maintaining adequate oxygen levels can be complex.
This can be mitigated by implementing controlled ventilation strategies and using selective membranes for CO2 removal can enhance process efficiency.
Agricultural residues:
Rice bran: Rich in nutrients and widely available, rice bran is commonly used for enzyme production.
Wheat bran: Another nutrient-rich substrate, wheat bran is used for producing various enzymes and organic acids.
Food processing wastes:
Fruit peels: Rich in sugars and fibers, fruit peels are ideal substrates for producing biofuels and bioactive compounds.
Vegetable wastes: These wastes can be utilised for producing biofertilizers and biopesticides.
Lignocellulosic biomass:
Sugarcane bagasse: A by-product of sugar production, bagasse is used for bioethanol production.
Corn stalks: These are used for producing biofuels and biochemicals through SSF.
Various microorganisms, including bacteria, yeasts, and fungi, are employed in SSF. The choice of microorganism depends on the desired product and the characteristics of the substrate.
Fungi:
Filamentous fungi, such as Aspergillus niger and Rhizopus oryzae, are commonly used in SSF due to their ability to grow on solid substrates and secrete hydrolytic enzymes.
Fungi are particularly effective in breaking down complex polysaccharides in lignocellulosic materials.
Bacteria:
Bacterial species such as Bacillus and Lactobacillus are used for the production of enzymes and organic acids.
Bacteria are less commonly used in SSF due to their higher moisture requirements but can be effective when conditions are optimised.
Strain optimisation:
Genetic engineering techniques are used to enhance the metabolic capabilities of microbial strains. This includes modifying metabolic pathways to increase product yield and tolerance to environmental stresses.
Recombinant DNA technology:
Introduction of foreign genes into microbial genomes can enable the production of novel bioproducts. For example, engineering Aspergillus niger to express recombinant enzymes for industrial applications.
Adaptive evolution:
Microbial strains are subjected to selective pressures in controlled environments to enhance their performance. This process, known as adaptive evolution, can lead to the development of robust strains with improved efficiency.
NIRAS, with its extensive experience in SSF, is a leading collaboration partner in designing process equipment and optimising fermentation processes, ensuring high efficiency and sustainability in industrial applications.